Why 2X Earth size is routinely sold out
Astronomers look to solve the mysteries of why some planets are a lot more rare than others
November 21, 2022 | Written by Jackie Appel
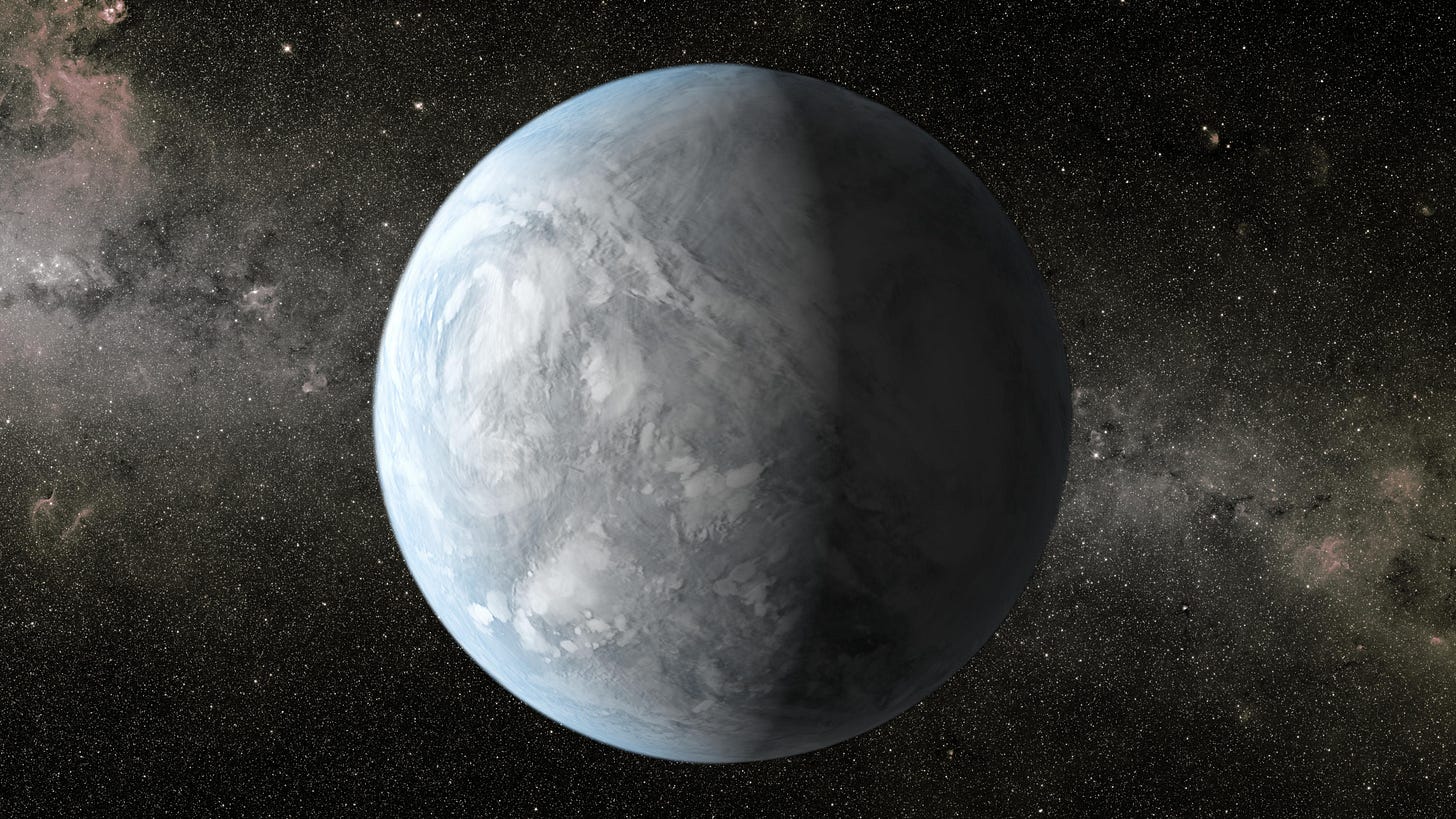
There’s a lot we don’t know about exoplanets. Despite crossing the “5,000-exoplanets-confirmed” threshold fairly recently, we have only just begun mapping the planets of the broader universe.
But even with our limited data set, the mapping we have managed to accomplish has already begun to introduce some entirely new mysteries ripe for further investigation. One of the bigger questions that has now popped up is this: there is a whole category of planets that we expected to exist — but it just doesn’t seem to be there.
Planets about 2 times larger than Earth seem to be abnormally rare amongst planetary systems. We have spotted plenty of super-Earths (supposedly rocky planets about 1.4 times the size of Earth) and plenty of mini-Neptunes (supposedly water-rich planets about 2.5 times the size of Earth), but planets in the middle are in short supply. This creates what is known as the “radius valley.”
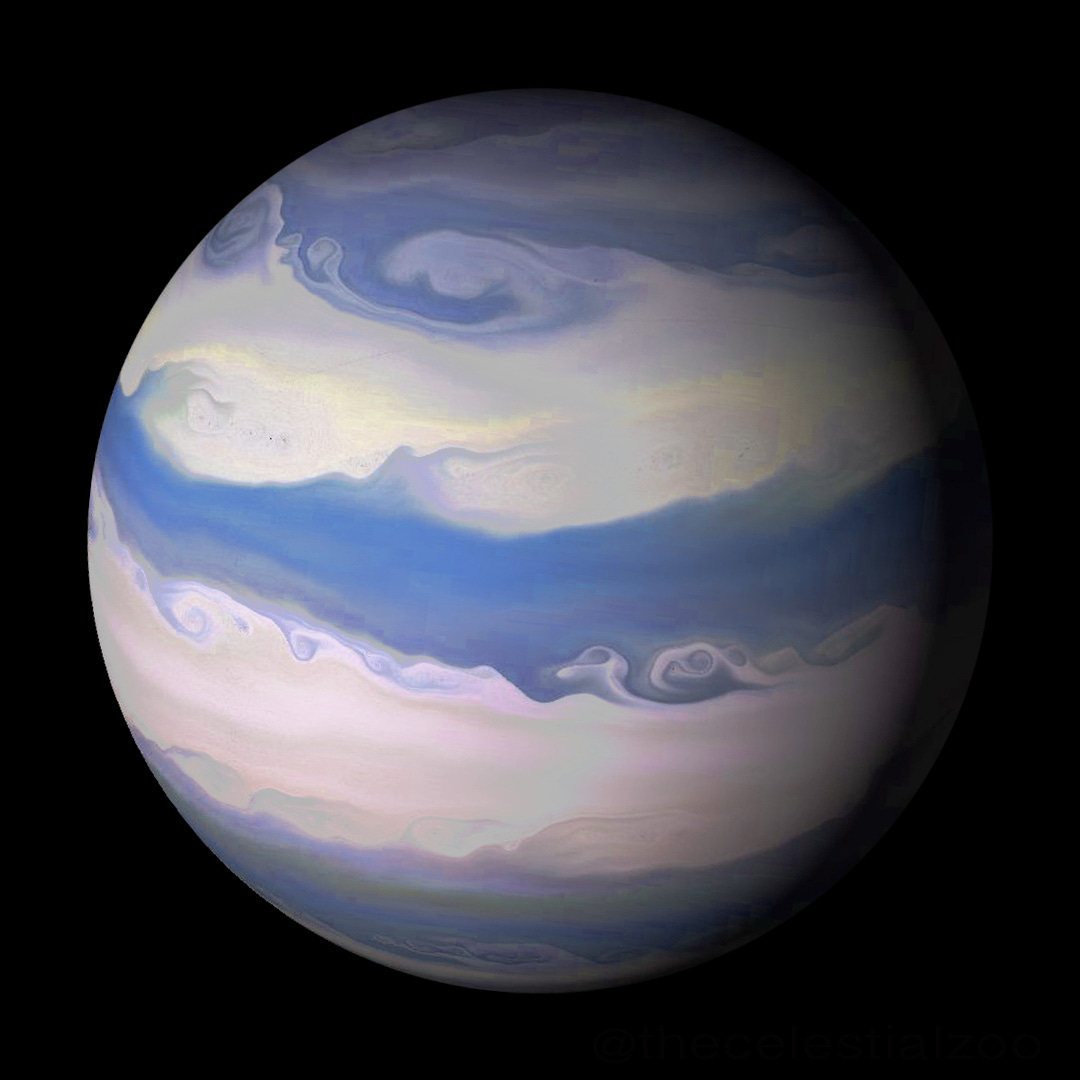
The existence of the radius valley has been a puzzle for quite some time in the exoplanet community. We’ve known enough to be sure it exists, but not to know why. Recently, however, a team led by researchers from Rice University proposed a possible explanation – planetary migration, the slow drifting of planets off their orbital path.
While we know that planets don’t just appear around a star fully-formed, there is still a lot we don’t know about planetary formation. One thing we do know, however, is that the process starts in what is called a protoplanetary disk. This is a disk of dust and gas that surrounds a star early in its life.
As the particles in this disk orbit the star and bump around, gravity starts to take hold, and the dust and gas begins forming into little clumps. Through collection and collision, these little lumps get bigger and bigger until they form into planets.
Frequently, these planets that form in the early days of a system fall into some kind of resonance pattern with each other — a pattern of orbits where for every time one planet orbits its sun once, another may orbit twice, another may orbit 4 times, and so on. But though they may reach these neat, musical patterns very quickly, they often don’t stay there for long.
Not all of the dust gets swept up into mini-Neptunes and super-Earths at the same time. “When these planets are forming, they are still in the disk,” said André Izidoro, lead researcher on the project. “So, the planets are migrating because of gravitational interactions with the gas.”
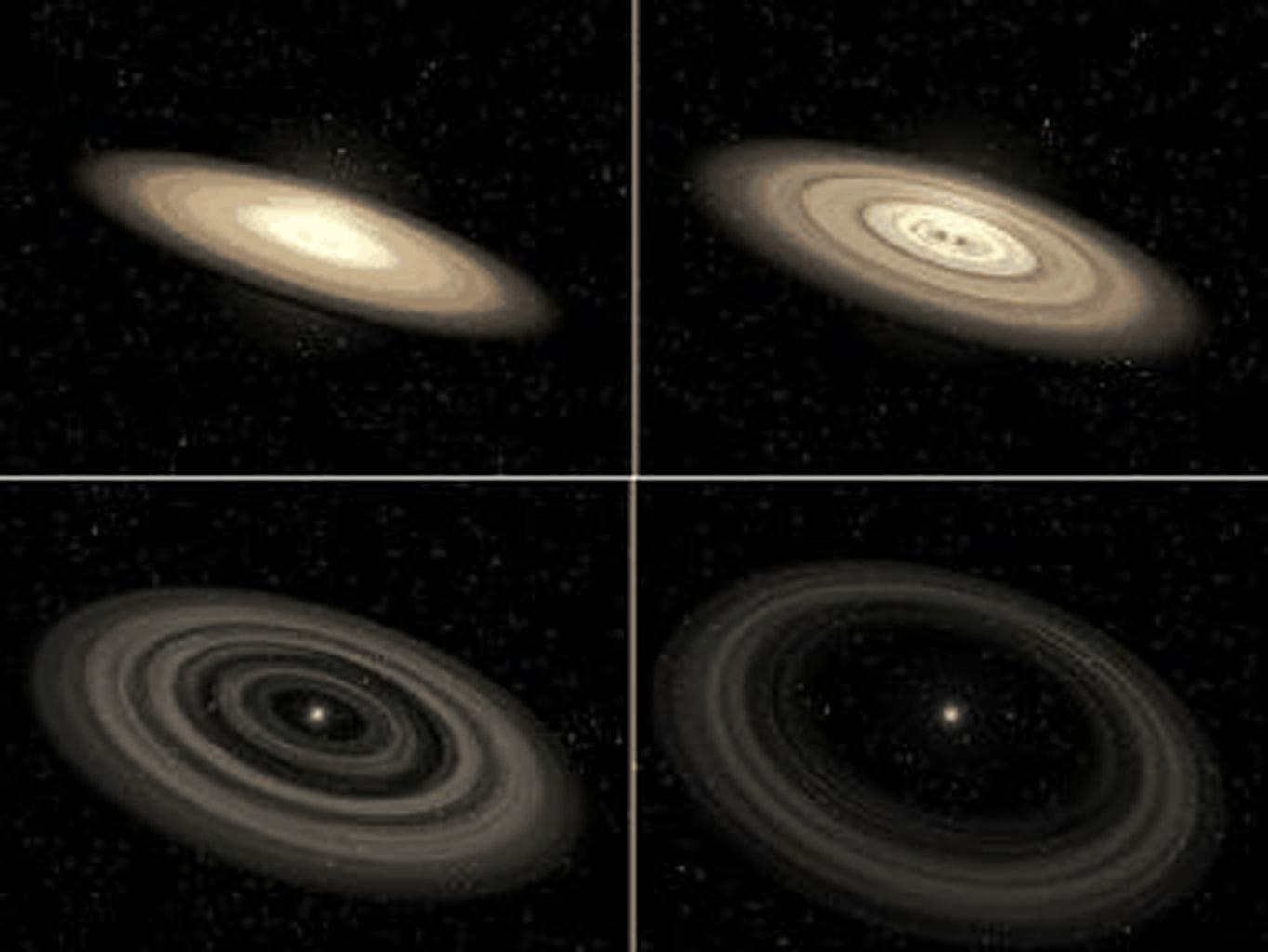
Using detailed computer models, the team simulated what happens when the gas starts affecting the movement of the planets. And they found two major consequences. The first, is that many planets collected puffy hydrogen-based atmospheres, often fluffing up to double their original radius.
The second, is that the debris started to cause planetary drift — tugging and pulling on the planets in their harmonious orbits, and slowing them down so that they fall out of resonance and start to drift inwards towards the star. As they drift inward, they often cross paths, resulting in collision.
And while that might sound a little cosmically dramatic, collision in early solar system formation is actually incredibly common. It’s likely why the Earth looks like it does, and why we have our Moon. But that doesn’t mean it isn’t responsible for radically reshaping things.
The team followed their model through the collisions of three major combinations — super-Earth and mini-Neptune, two super-Earths, and two mini-Neptunes — to see how these drift-triggered collisions affected overall planetary formation in their simulated systems.
The first thing they noticed was that all three scenarios resulted in the puffy hydrogen atmospheres being blasted off into space, shrinking the planets’ radii significantly. The second major takeaway was that no matter what collision they simulated, it fell on one side or another of the radius valley.
If two super-Earth’s collided, the resulting planet was about four times our Earth’s mass. But having lost its puffy atmosphere and been compressed due to increased internal gravity, it remained about the size of one super-Earth, thus falling below the radius valley. On the flip side, if two mini-Neptunes collided, the resulting planet was about eight times the size of our Earth. And because water is less dense than rock and so subject to comparatively less internal gravity, the planet doesn’t compress down very far and stays above the radius valley. If a super-Earth hits a mini-Neptune, it still ends up a watery world and stays above the valley.
Interestingly, this model only works if one thing is true — that mini-Neptunes are in fact watery planets. Our Neptune is what we call an ice giant, meaning it is mostly made of water, ice, and gas. This sets it apart from gas giants like Jupiter that are made mostly of hydrogen and helium, and apart from rocky planets like Earth that are mostly made of rocks and metals. Some astronomers believe both super-Earths and mini-Neptunes are rocky planets. But in showing that watery planets would have to be part of the formation story of the radius gap, Izidoro and his team believe they have backdoored their way into proving that mini-Neptunes are like our own Neptune in more than just size and name. “If you start with only rocky planets, you fill the gap, because you tend to have multiple collisions,” said Izidoro.
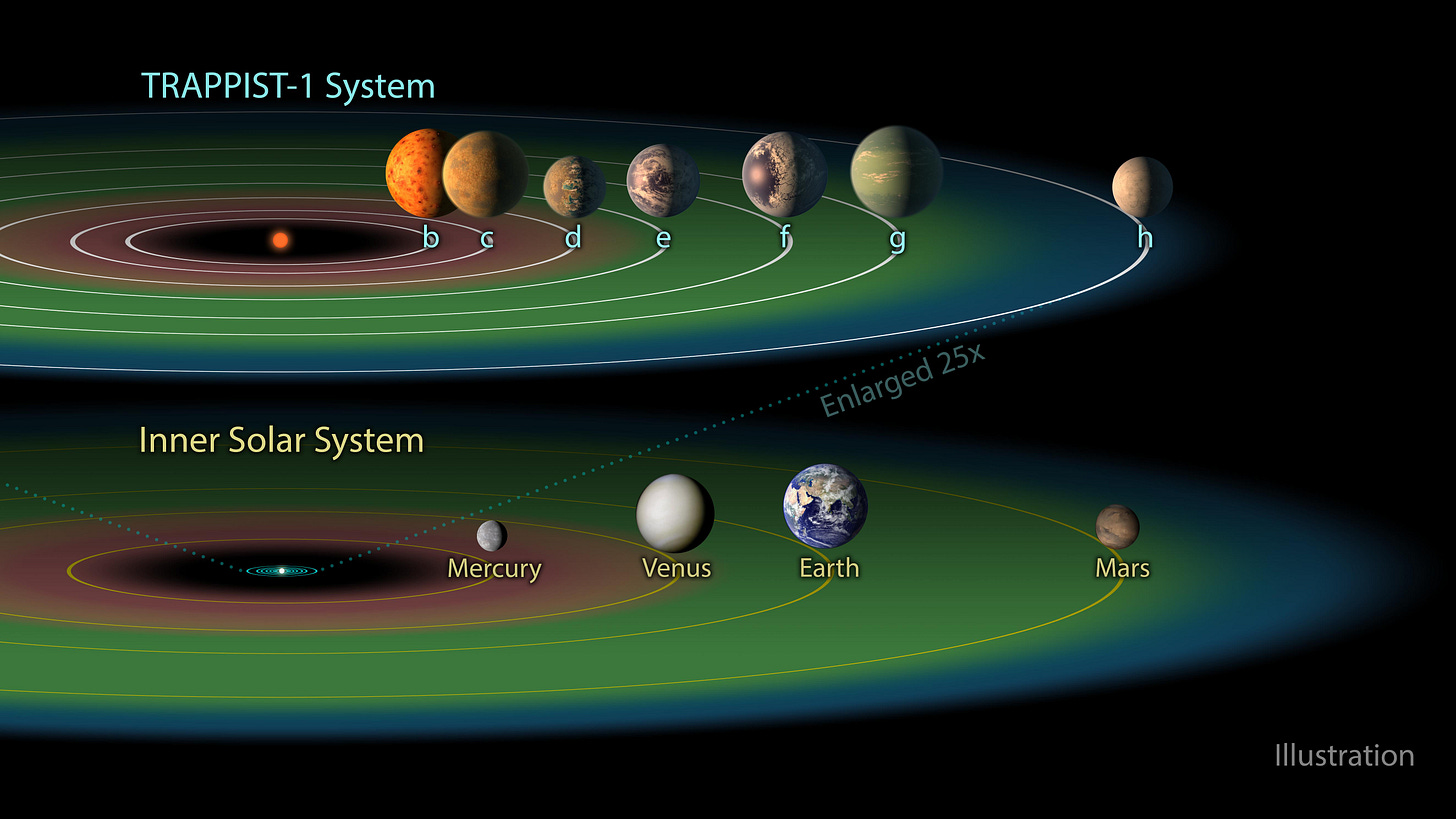
And on top of that, the team believes their work can help explain yet another mystery in the exoplanet world — the “peas in a pod” phenomenon. This is the idea that the inner planets of a system all tend to look the same, much like our own Mercury, Venus, Earth, and Mars all look pretty similar. This would be an extremely likely outcome of the planetary drift and subsequent collisions described by Izidoro and his team. All the smaller inner planets would end up as rocky planets after they were finished being all smashed up, leaving the bigger watery planets to hang out further from the sun where the water doesn’t all just burn away.
Lots of observation over a very long time will have to happen before we can observationally confirm or deny this proposal. But the idea makes sense, and has serious potential for explaining one of the big mysteries of both exoplanets and planetary formation. As new data comes rushing in every day, we hopefully won’t have to wait too much longer to know even more.
Meet the Writer
Jackie Appel is a freelance writer for Continuum with a degree in astrophysics from Ohio State University and a master’s degree in Science, Health and Environmental Reporting from New York University. She has previously written for Scienceline and loves sharing the weird wonders of the universe with anyone who wants to listen. Jackie thinks “spaghettification” is the best science word ever (and a very cool phenomenon!).